The Evolution of Pollination as an Ecosystem Service by Bee Redfield, Pollinator Partnership
October 29, 2024
Locust borer beetle (Megacyllene robiniae) on goldenrod (Solidago), Beatriz Moisset (US Forest Service)
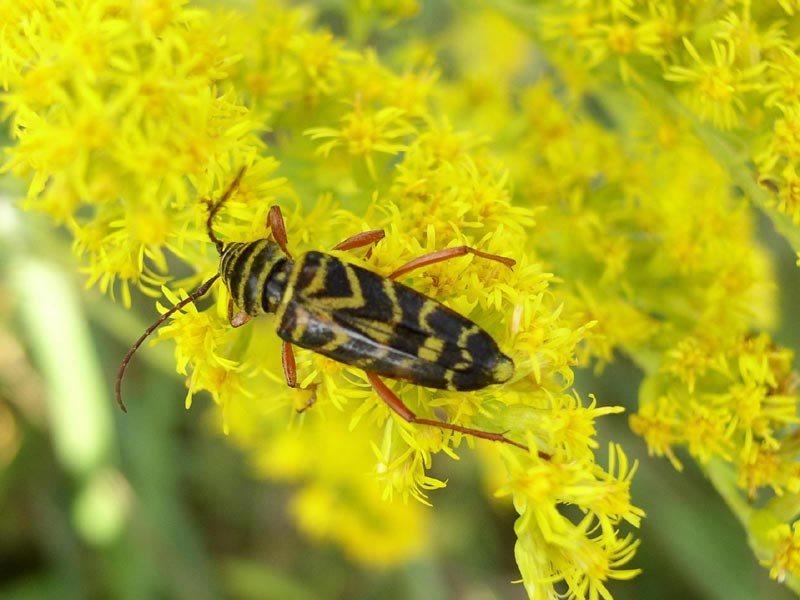
Pollination is a fundamental ecological process that enables the reproduction of flowering plants, or angiosperms, which constitute most plant species on Earth. This process has evolved over millions of years, transforming into a critical ecosystem service performed by various animals. Here, we explore the evolution of pollination, the diversity of pollinators, and the significance of this service in maintaining healthy ecosystems around the world.
The origins of pollination trace back to the early Cretaceous period, approximately 130 million years ago, when the first flowering plants (called angiosperms) appeared. These plants developed mutualistic relationships with insects. As plants and their pollinators coevolved, flowers began to develop traits that attracted specific pollinators, such as vibrant colors, enticing scents, and nectar rewards. These traits are known pollinator syndromes.
Did you know that the first-ever angiosperm pollinators were beetles?
Lichnomesopsyche gloriae (a type of ancient scorpionfly) from late middle Jurassic period China, Wenying Wu (Smithsonian Institution)
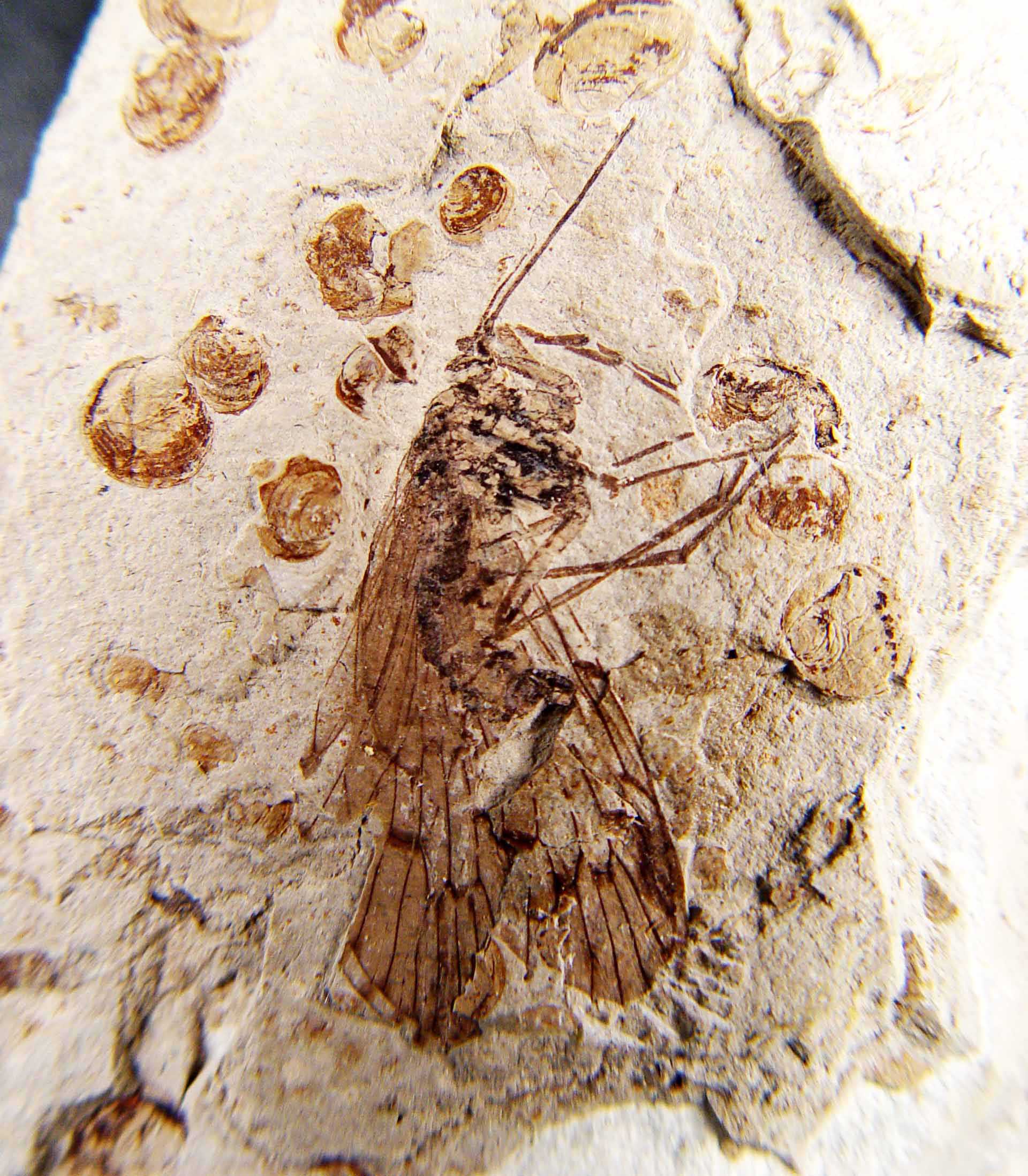
Scientists believe that beetles were the primary pollinators of early flowering plants. The exact genus and species of these early pollinators are not precisely known due to the limitations of the fossil record. However, it is hypothesized that these ancient beetles, which belonged to early Coleoptera groups, were already feeding on pollen and other plant parts long before flowering plants appeared. As flowering plants emerged, they co-opted these beetles into their reproductive strategies. The development of mutualistic relationships with beetles allowed flowering plants to enhance their reproductive success by ensuring efficient pollen transfer (Thien, 1980). More recent scientific findings have also demonstrated that scorpionfly (Mecoptera) species likely developed an adapted mouthpart to provide pollination services to early gymnosperm species (non-flowering plants with exposed seeds, such as conifers). These scorpionfly pollinators became extinct during the late Cretaceous period (approximately 105 million years ago), just as angiosperm pollinators like beetles and butterflies began to appear (Ren et al., 2009).
The coevolution between flowering plants and their pollinators led to an incredible diversification of both groups
An ornate day gecko (Phelsuma ornata) drinks nectar from tree blossoms in Mauritius, Mark W. Moffett (National Geographic)
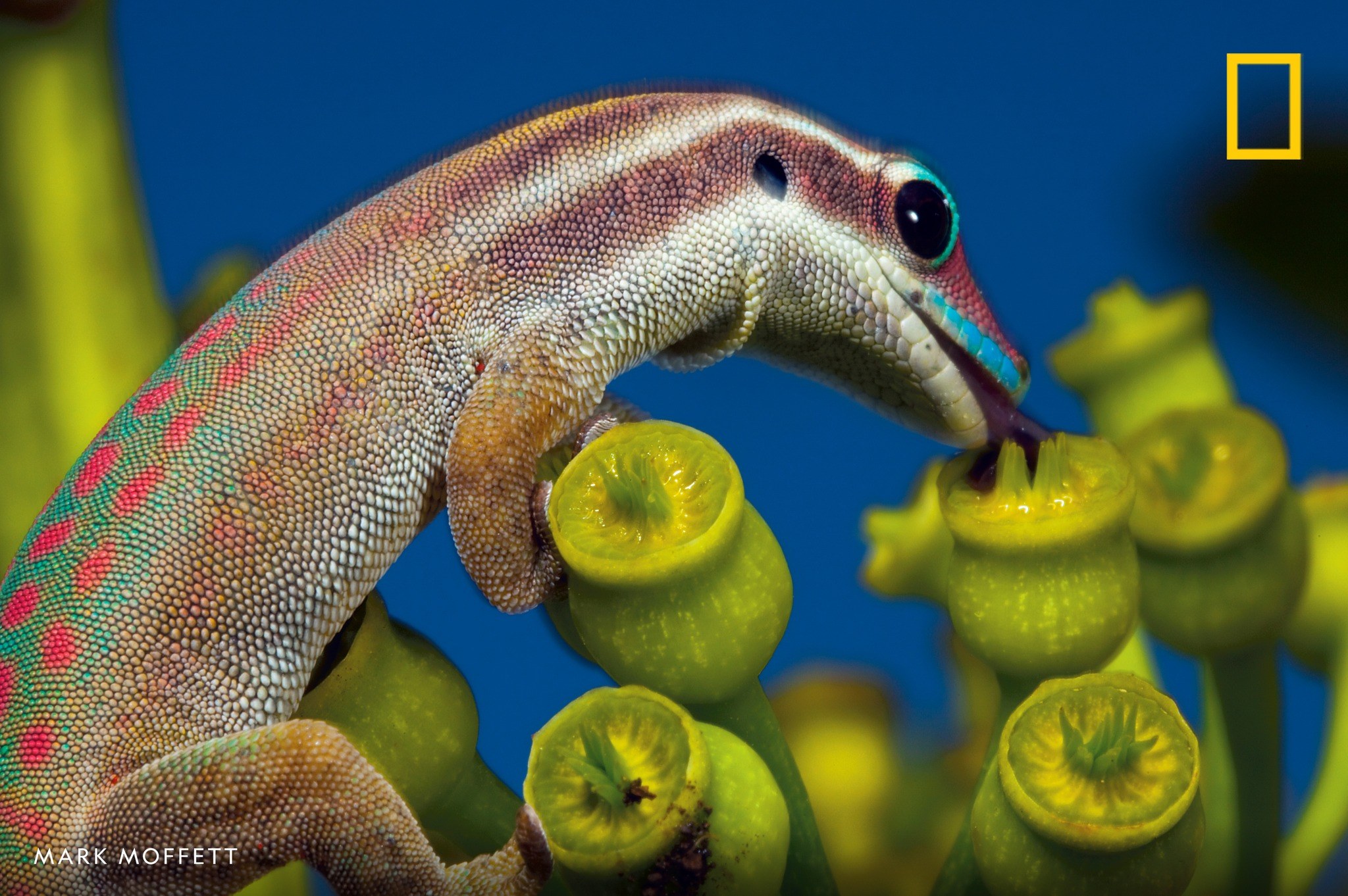
Plants evolved various strategies to attract and reward pollinators, such as producing nectar and developing bright, enticing flowers. Pollinators, in turn, developed adaptations to efficiently access floral resources. This mutualistic relationship created a win-win scenario: plants could produce less pollen since pollinators ensured precise delivery to the target flowers, unlike non-pollinator-dependent plants. Meanwhile, pollinators gained access to nutritious pollen and nectar, essential to their survival. This mutualistic coevolution resulted in the emergence of the following distinct pollinator syndromes (and many more!):
1. Insect Pollinators
- Bees: Bees are perhaps the most well-known pollinators, with their hairy bodies adapted to collect and transfer pollen. They are particularly attracted to flowers with bright colors and sweet scents (Ollerton, Winfree, & Tarrant, 2011).
- Butterflies and Moths: These insects are drawn to flowers with landing platforms and deep corollas. Butterflies are active during the day, while moths typically pollinate nocturnal flowers (Ollerton et al., 2011).
- Beetles and Flies: These early pollinators are often attracted to flowers with strong, sometimes unpleasant, odors that mimic decaying matter (Ollerton et al., 2011).
2. Bird Pollinators
- Hummingbirds: Found primarily in the Americas, hummingbirds are attracted to tubular, brightly colored flowers. Their long bills and specialized tongues enable them to extract nectar while transferring pollen (Potts, Imperatriz-Fonseca, & Ngo, 2016).
- Honeycreepers and Sunbirds: In regions like Hawaii and Africa, these birds have evolved to feed on nectar from specific flowers, playing a crucial role in their pollination (Potts et al., 2016).
3. Mammal Pollinators
- Bats: While many bats are insectivores, some are important nocturnal pollinators, especially in tropical and desert ecosystems. They are attracted to large, white or pale flowers that emit strong fragrances at night (Potts et al., 2016).
- Small Mammals: Some small mammals, like rodents and marsupials, also contribute to pollination by feeding on nectar and subsequently transferring pollen from plant to plant (Potts et al., 2016).
4. Other Pollinators
- Reptiles: In some ecosystems, lizards and geckos can act as pollinators by feeding on nectar and inadvertently transferring pollen collected on their bodies to other plants (Ollerton, 2017).
- Humans: While not a natural pollinator, human activities such as agriculture and horticulture often involve the manual pollination of crops and ornamental plants (Ollerton, 2017).
Over time, both plants and their pollinators have developed specialized adaptations to enhance the efficiency of pollination. These adaptations include:
- Floral Morphology: As mentioned above, plants have evolved diverse flower shapes, sizes, and colors to attract specific pollinators. For example, deep tubular flowers are suited for hummingbirds, while flat, open flowers cater to bees (Ollerton et al., 2011).
- Nectar Guides: Many flowers have visual, or UV patterns known as nectar guides, which direct pollinators to the nectar, ensuring effective pollen transfer (Ollerton et al., 2011).
- Pollen Presentation: Some plants have developed mechanisms to control pollen release, such as the explosive pollen release in certain orchids or the gradual presentation in sunflowers (Ollerton et al., 2011).
- Pollinator Fidelity and Constancy: Many pollinators, such as bees, exhibit floral fidelity, meaning that they consistently visit the same species of flower during a foraging trip. This constancy increases pollination efficiency by maximizing the likelihood of pollen transfer between flowers of the same species, enhancing reproductive success and reducing cross-species pollination loss (Waser, 1986).
- Buzz Pollination: Some plants, like tomatoes, have poricidal anthers that release pollen only when vibrated at a specific frequency, a process known as buzz pollination. Bees (especially bumble bees) perform this behavior by vibrating their wing muscles, which shakes the pollen loose and ensures efficient transfer to other flowers.
A white-lined sphinx moth or “hummingbird moth” (Hyles lineata) nectaring on Rocky Mountain beeplant (Cleomella serrulata) in the Seedskadee National Wildlife Refuge, Tom Koerner (USFWS)
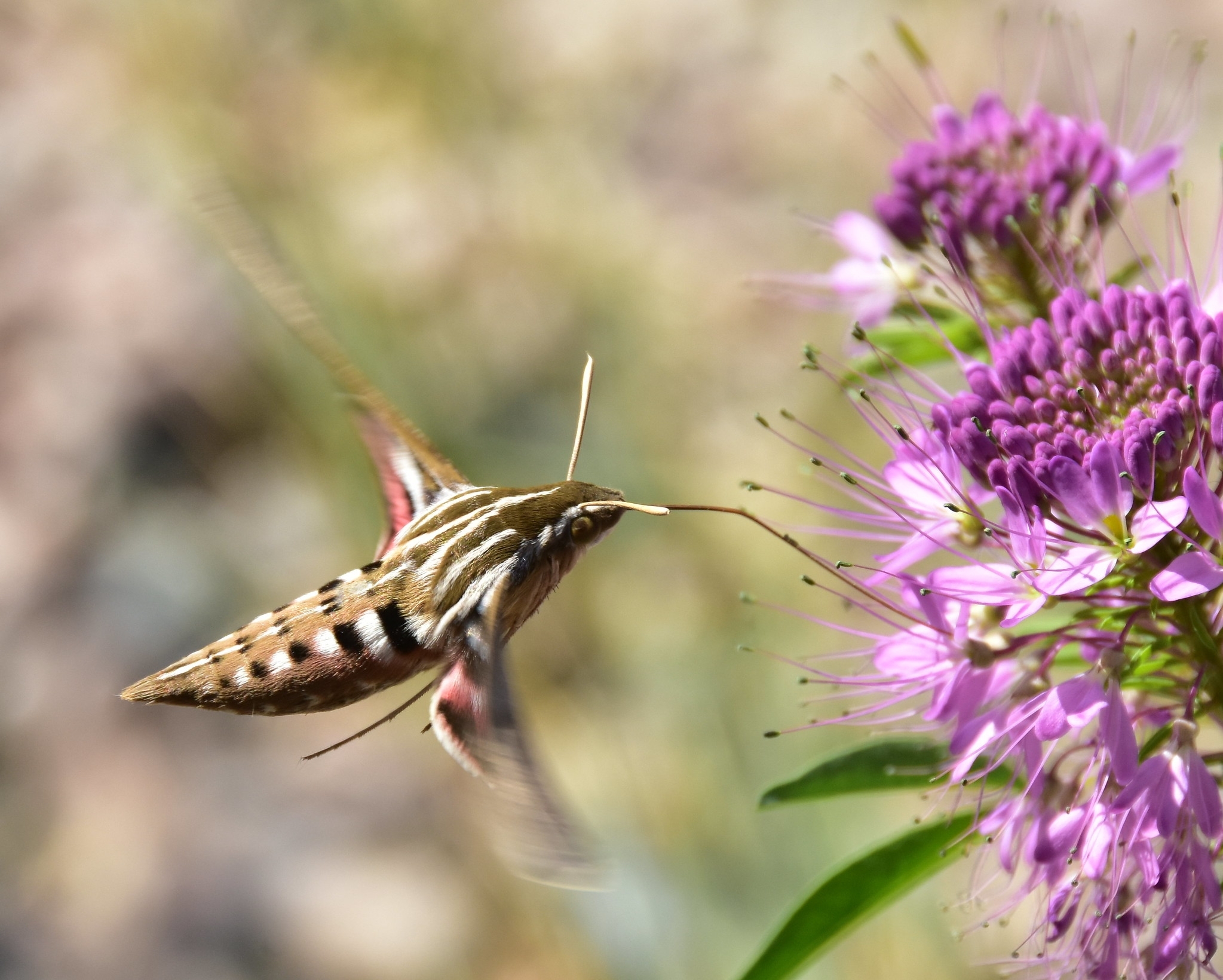
Bees are now considered among the most efficient and important pollinators, but you may be surprised to learn they evolved from wasps!
American ginseng (Panax quinquefolius), native to the Eastern United States and pollinated by sweat bees and hoverflies, has been used in medicines for hundreds of years (USFWS)
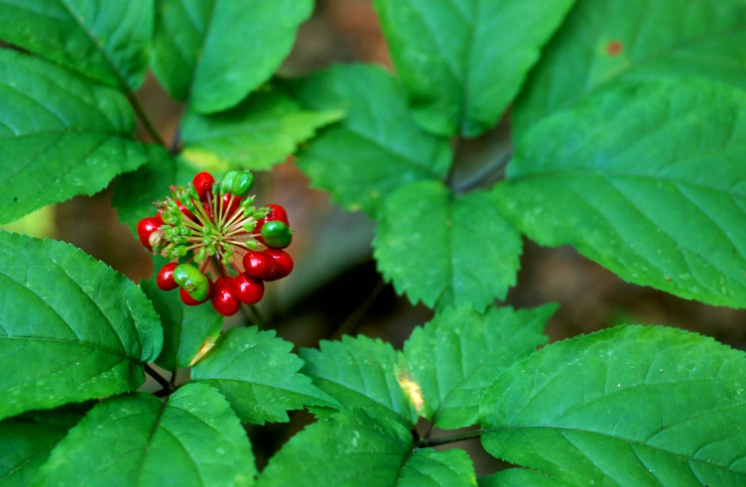
Scientists hypothesize that bees evolved from wasps around 100 million years ago. The wasps that gave rise to bees were likely predators that fed on other insects. Over time, some of these wasps began to feed on pollen and nectar instead of hunting other insects. This dietary shift led to the evolution of physical adaptations such as branched body hairs for collecting pollen and the development of structures like the pollen basket (corbicula) on their legs (Danforth, Sipes, Fang, & Brady, 2006). This shift also helped to drive the diversification of flowering plants, which now include 300,000 species worldwide. Bees themselves have diversified into approximately 20,000 species, contributing to complex webs of interaction between plants and pollinators that sustain ecosystems today (Danforth et al., 2006; Ollerton et al., 2011).
Pollination as an ecosystem service is now vital for the reproduction of many plants, including a significant portion of the world’s crops. Approximately 75% of global food crops depend on animal pollination, making it essential for food security and agriculture (National Research Council, 2007). Also, pollination supports biodiversity by enabling plant reproduction, which sustains other wildlife that depend on these plants for food and habitat (Potts et al., 2016).
Moreover, the plants that benefit from pollination contribute to essential ecosystem services that humans depend on daily. These plants play critical roles in carbon sequestration, helping to mitigate climate change; they also contribute to water purification and soil stabilization, protecting against erosion and improving water quality (Daily & Matson, 2008). Pollinated plants also provide the raw materials for medicines, textiles, and countless other products that support our economies and quality of life (Pimentel et al., 1997). The ripple effect of pollination extends far beyond the flowers and fruits we see – it supports the health and functionality of entire ecosystems, upon which all life depends.
However, human activities have significantly impacted pollinator populations and their habitats. Pesticide use, habitat destruction, climate change, and the introduction of invasive species threaten pollinators worldwide. Conservation efforts are crucial to protect these vital organisms and the ecosystem services they provide. Strategies include creating pollinator-friendly habitats, reducing pesticide use, and supporting research and public awareness initiatives (National Research Council, 2007).
The evolution of pollination as an ecosystem service is a remarkable demonstration of the intricate relationship between plants and animals. From ancient beetles to modern-day hummingbirds and bees, pollinators play a crucial role in sustaining ecosystems and agriculture. By understanding and preserving these relationships, we protect the health and diversity of our planet’s flora and fauna, and ourselves.
References
Daily, G. C., & Matson, P.A. (2008). Ecosystem services: From theory to implementation. Proceedings of the National Academy of Sciences, 105(28), 9455-9456. https://doi.org/10.1073/pnas.0...;
Danforth, B. N., Sipes, S., Fang, J., & Brady, S. G. (2006). The history of early bee diversification based on five genes plus morphology. Proceedings of the National Academy of Sciences, 103(41), 15118-15123. https://doi.org/10.1073/pnas.0604033103
National Research Council. (2007). Status of Pollinators in North America. Washington, DC: The National Academies Press. https://doi.org/10.17226/11761...;
Ollerton, J. (2017). Pollinators & Pollination: Nature and Society. Pelagic Publishing.
Ollerton, J., Winfree, R., & Tarrant, S. (2011). How many flowering plants are pollinated by animals? Oikos, 120(3), 321-326. https://doi.org/10.1111/j.1600-0706.2010.18644.x
Pimentel, D., Wilson, C., McCullum, C., Huang, R., Dwen, P., Flack, J., & Cliff, B. (1997). Economic and environmental benefits of biodiversity. Bioscience, 47(11), 747-757. https://doi.org/10.2307/1313097
Potts, S. G., Imperatriz-Fonseca, V. L., & Ngo, H. T. (2016). Safeguarding pollinators and their values to human well-being. Nature, 540(7632), 220-229. https://doi.org/10.1038/nature20588
Ren, D., C.C. Labandeira, et al. (2009. A probable pollination mode before angiosperms: Eurasian, long-proboscid scorpionflies. Science, 326(Nov. 6):840. https://www.science.org/doi/10...;
Thien, L. B. (1980). Patterns of pollination in the primitive angiosperms. Biotropica, 12(1), 1-13.
U.S. Fish & Wildlife Service. (n.d.). Pollinators. Retrieved from https://www.fws.gov/pollinators/
Waser, N.M. (1986). Floral constancy: Definition, cause, and measurement. The American Naturalist, 127(5), 593-603. https://doi.org/10.1086/284507...;